Diseases in a dish: Challenges of iPSC-based disease modelling
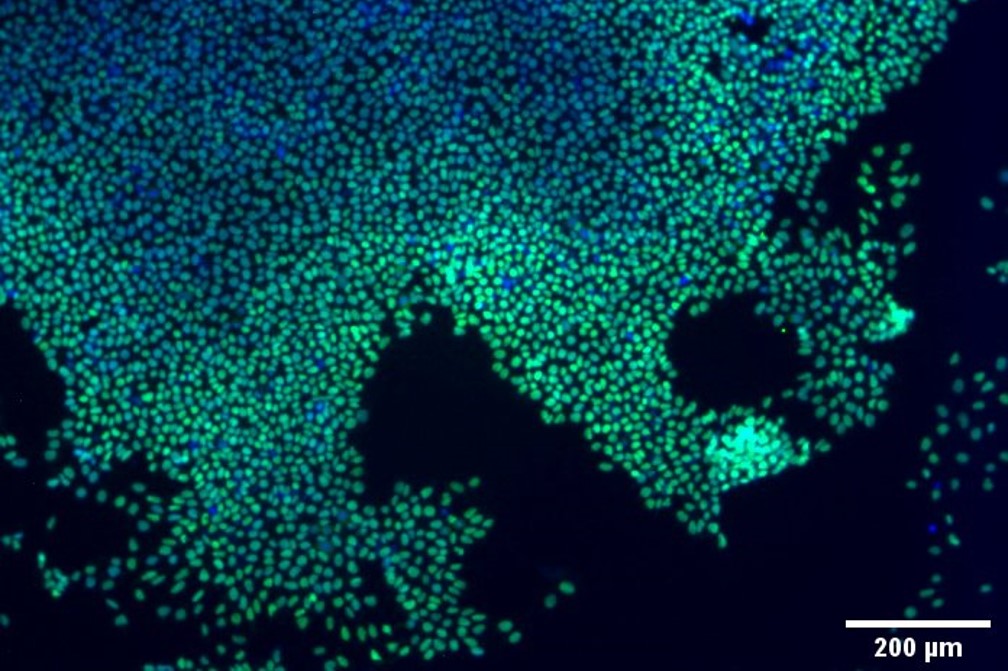
Understanding the mechanisms underlying human disease pathologies is essential for the development of therapeutic treatments. Developing a greater understanding of these mechanisms currently remains a challenging process due to the current lack of biologically relevant disease models.
The derivation of human induced pluripotent stem cells (iPSCs) from individuals suffering from a genetic disease, however, offers great new opportunities for basic research into specific syndromes and the development of therapeutic drugs, while overcoming conventional limitations of animal models for disease modelling and drug discovery. The breakthrough discovery in 2006 by Takahashi and Yamanaka paved the way in enabling the reprogramming of terminally differentiated, lineage-restricted adult somatic cells (such as skin fibroblasts, blood cells and other somatic sources) directly to a pluripotent state through the expression of the transcriptional factors: Oct4, Sox2, Klf, câ€myc, Nanog and Lin28. These iPSCs have the capacity for self-renewal and to give rise to any type of somatic cell type in the body. Patient-specific iPSCs can therefore provide unlimited disease-relevant cells in a personalized manner, serving as an extremely valuable resource for previously inaccessible cell types, including cardiomyocytes and neurons.
Despite iPSCs having potential in many biomedical applications, there are currently major challenges that need to be addressed to unleash the full potential of iPSCs for disease modelling, both in clinical settings and their use for safety pharmacology to provide more effective and safer regenerative therapy:
1) Maturity:
One of the critical problems encountered in using patient-specific iPSCs for in vitro disease modelling for drug discovery and safety pharmacology is the tendency of iPSC-derived cells exhibit immature functional characteristics similar to their respective embryonic or fetal-like phenotypic cells. Furthermore, many studies have reported heterogenous cell mixtures of varying proportions of phenotypic subtypes at the end of iPSC differentiation protocols. Therefore, modelling late-onset diseases remains a challenge since iPSC-derived relevant phenotypic cells lack both the adult maturation characteristics and specific cellular subtypes. There have been several approaches to induce the maturation of these primitive iPSC phenotypic cells, such as: Utilizing 3D co-culture to enhance paracrine-mediated stimulation of ageing and maturation, or the directed reprogramming of somatic cells such as fibroblasts into clinically relevant phenotypic cells such as neurons and cardiomyocytes to preserve the cellular ageing markers and, possibly, maturation state.
2) Genetic variation:
Studies of iPSCs derived from large populations have demonstrated variability in differentiated cell phenotypes and gene expression, which can be attributed to background genetic variation. Although cohorts of iPSC lines can be exploited to model human genetic heterogeneity, this effect can confound disease modelling in the absence of appropriate controls. Genetic correction of disease-causing mutations in patient-derived iPSCs should be used to generate otherwise isogenic control iPSCs for use as controls in disease models. The growing number of biobanks and iPSC repositories from which many iPSC lines for a particular disease are available will enable large scale studies to overcome the ‘signal-to-noise ratio’ in the phenotypic differences, especially for complex polygenic diseases.
3) Genome stability:
iPSCs are susceptible to genetic instability and, since these cells are maintained in culture for prolonged periods of time, can accumulate chromosomal abnormalities, copy number variants and loss of heterozygosity over a period of time in vitro culture and expansion. There are also concerns around genome instability and epigenetic memory associated with the reprogramming process and iPSC maintenance. These pose challenges to the integrity of iPSC derivatives and the modelling of diseases that are epigenetically influenced by environmental factors. Therefore, adequate measures need to be devised to eliminate the chromosomally abnormal cells and cells with genetic alterations which may undermine the integrity of experimental data.
iPSC-based disease modelling has proven to be vital in both biomedical research and personalized regenerative medicine, as shown in the past decade. iPSCs are, capable of unravelling novel pathological mechanisms of many diseases at the cellular level and are also showing potential in ongoing and emerging clinical trials with iPSC-derived cells. iPSC-based therapeutic approaches are, however, still in their early stages and several obstacles need to be overcome for effective translation into clinical settings with uncompromised patient safety.
Technological advances in combining iPSC technology and 3D multicellular organoids (such as personalized organ-on-chip technology) with gene editing, artificial intelligence and high throughput ‘omics’ methodologies, will eventually be used for high-throughput drug discovery. This may provide us with a better understanding of the complex mechanisms underlying pathological cellular behaviour, which will also contribute to the adoption of iPSC-based therapeutic applications in the future.
Cell Guidance Systems offers a karyotype screening service that can identify chromosomal abnormalities, copy number variants and loss of heterozygosity.
IMAGE: Nanog stained human iPSCs, Cell Guidance Systems