ELMs: Engineered living materials adapting to life
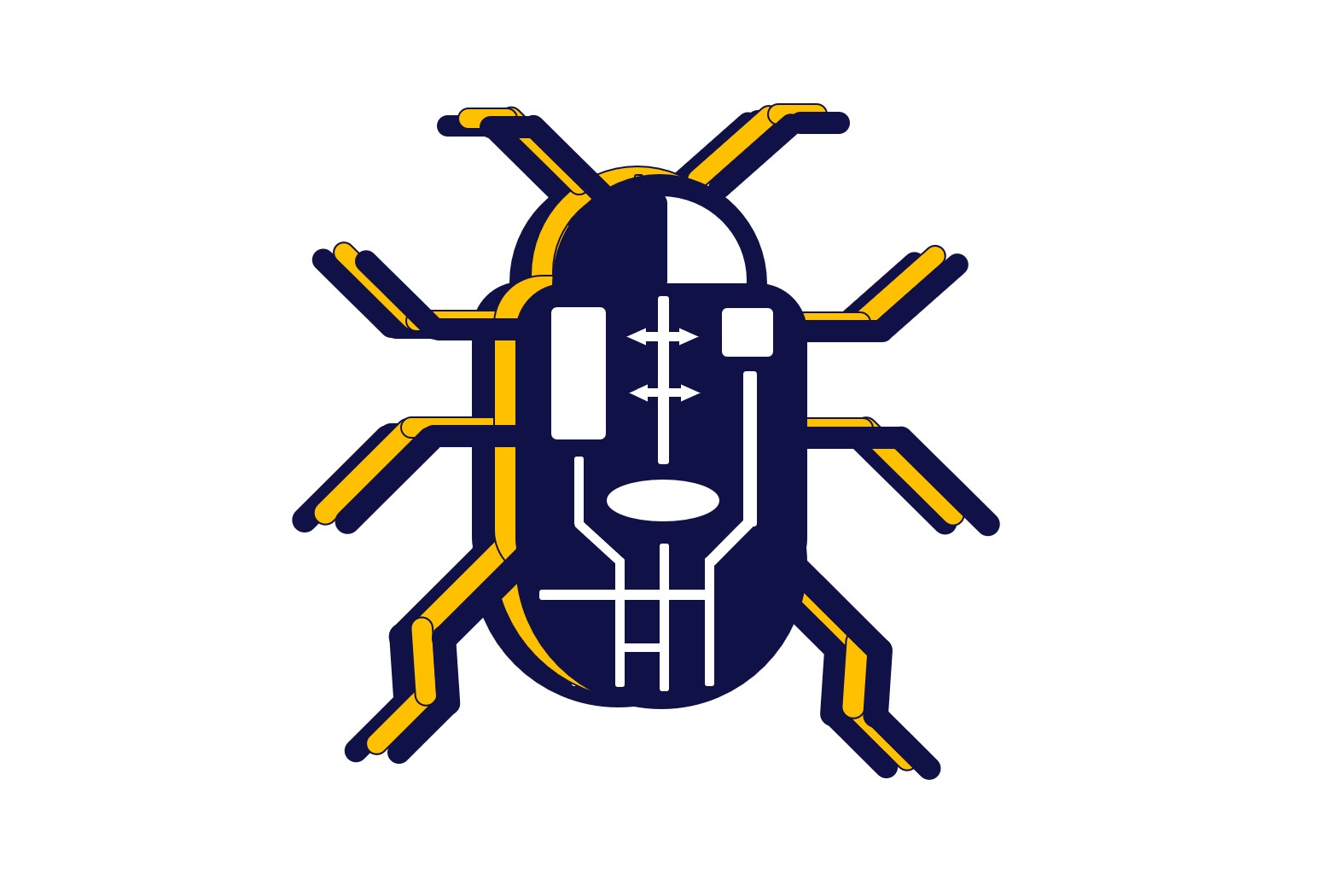
Living cellular structures that can respond to their environment are being developed. These structures seek to revolutionise the methods of traditional material technology and offer ways to address real-life challenges in medicine, biotechnology and sustainability.
ELM objectives
A long-term objective in the field of tissue engineering is to create a material system that possesses the unique advantages of biological systems, including an ability to grow, regenerate, evolve and adapt. Thus far, no designed biomaterials, either biological or synthetic, have been able to fully recapitulate these properties. To bridge this technology gap, a rich area at the interface of synthetic biology and materials science is creating engineered living materials (ELMs) that can, to some extent, take on the properties of natural living materials.
What are ELMs?
The field of ELMs overlaps with regenerative medicine but also addresses non-regenerative applications. It capitalises on the design of bioengineered cellular structures functioning as living systems autonomously and sustainably. A key difference between ELMs and other engineered structures is that ELMs specifically aim to exploit the role of living cells as nanomaterial factories that can constantly sense and draw upon diverse energy feedstocks from their environment. This harnessed energy is then used by the cells to play an active role in the tasks of material synthesis, and self-organisation into hierarchically defined structures and subsequent maintenance/modulation of the structure according to cellular responses. ELMs rely on a variety of forms, such as genetic engineering of the cells, alteration of chemical/material properties to coordinate control over metabolic processes and spatial/mechanical patterning to position the cells.
Bacterial ELMs
Many of the potential applications of ELMs are not focussed on regenerative medicine. This has enabled the use of bacteria to create ELMs: bacteria proliferate rapidly, are genetically tractable and secrete a complex assortment of extracellular matrix components. Bacterial biofilms, in particular, have attracted interest as readily programmable scaffolds for the construction of ELMs. By controlling factors like strain, spore formation, biofilm composition and initial bacterial/additives concentration, it is possible to endow programmability and desired responses to the materials. To further push the boundaries of ELMs’ capabilities, these microorganisms can also be grown, assembled, mixed or layered synergistically with externally provided abiotic material (polymers, carbons, metals, etc.) to create biocomposites. One developed application is a mercury-sequestering microbial filter.
Eukaryotic ELMs
Whilst significant progress has been made with bacterial ELMs, it’s been more challenging to control the behaviour of eukaryotic cells. An ELM has been developed using yeast which utilized mixtures of genetically modified Saccharomyces cerevisiae. The yeast cells were engineered with different novel surface molecules which interacted with each other to generate the complex structures. ELMs for regenerative medicine will be even more challenging as they will necessitate the use of even more complex mammalian cells.
Future ELM applications
The possibilities for ELMS are almost endless. Ongoing efforts across many labs to design ELMs include responsive gels containing antibiotic-producing spores, smart self-cleaning surfaces that can metabolise food spills and self-healing biological concrete. There are also a few commercially successful examples of ELMs, such as ‘tree shaping’ structures, mycelial materials for waste reduction/recycling, and bacterial cellulose paper. These ELMs also serve as prime examples of sustainable materials, as users don’t need to consider energetic needs and the cells are fully recyclable throughout their life cycle.
Progress to date in the nascent ELMs field has been made in small, laboratory-scale production. Significant advances in engineering principles and molecular knowledge will be needed to fully support the true integration of ELMs into everyday life. These living systems also need to be further studied to optimise the long-term robustness, responsivity, viability, scalability and overall safety of the ELMs in macroscopic structures.