Hydrogels for 3D Bioprinting
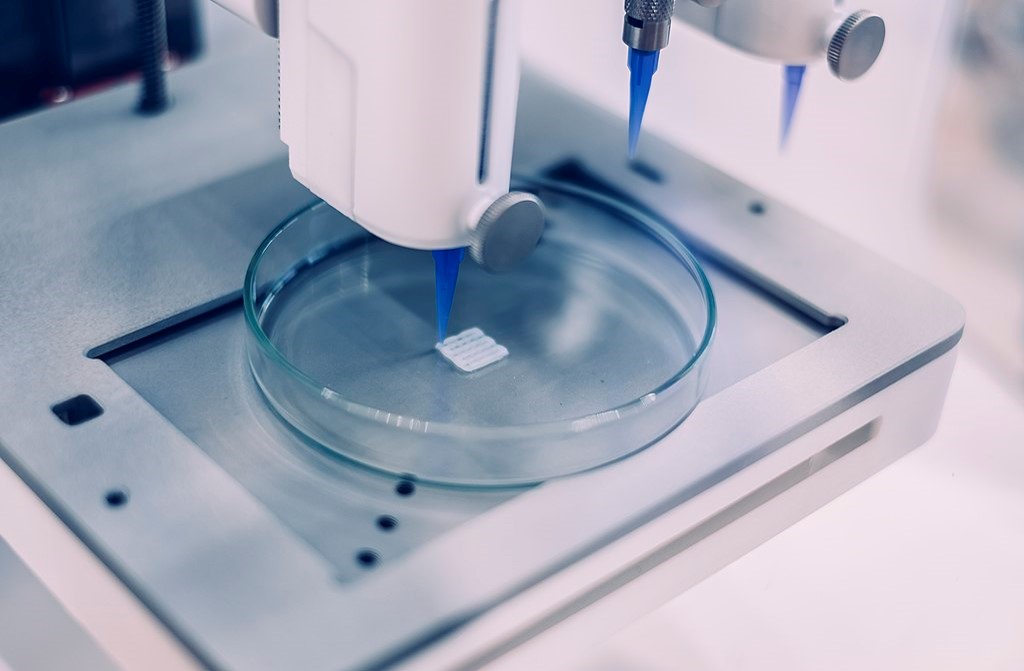
Three-dimensional (3D) bioprinting is an emerging technology that enables the fabrication of complex, biomimetic tissue constructs for applications in tissue engineering, regenerative medicine, and drug testing. Hydrogels, which are hydrophilic polymeric networks capable of absorbing large amounts of water, have emerged as promising bioinks for 3D bioprinting due to their biocompatibility, tunable mechanical properties, and ability to support cell adhesion, proliferation, and differentiation.
Types of Hydrogels for 3D Bioprinting
A wide range of hydrogels have been developed from natural and synthetic materials.
Natural Hydrogels
Natural hydrogels are derived from biological sources and often possess inherent bioactivity and biocompatibility, making them suitable for 3D bioprinting applications Examples of natural hydrogels include alginate, chitosan, gelatin, hyaluronic acid, and fibrin. These hydrogels can be modified to improve their printability, mechanical properties, and bioactivity, such as by crosslinking or blending with other polymers.
Synthetic Hydrogels
Synthetic hydrogels are chemically synthesized polymers that can be tailored to have specific properties, such as mechanical strength, degradation rate, and bioactivity. Examples of synthetic hydrogels include poly(ethylene glycol) (PEG), poly(vinyl alcohol) (PVA), and poly(acrylic acid) (PAA). More complex synthetic hydrogels, such as Peptigel, contain components (peptides) seen in natural matrices. Synthetic hydrogels can be functionalized with bioactive molecules, such as growth factors and cell adhesion peptides, to enhance their ability to support cell growth and tissue regeneration. PODS growth factors ensure that the growth factor is stabilized within the hydrogel ensuring sustained availability.
Hybrid Hydrogels
Hybrid hydrogels combine the advantages of both natural and synthetic hydrogels, such as the bioactivity of natural hydrogels and the tunable properties of synthetic hydrogels. Examples of hybrid hydrogels include gelatin-methacryloyl (GelMA), which is a photocrosslinkable derivative of gelatin, and poly(ethylene glycol)-fibrinogen (PEG-Fb), which is a synthetic-natural hybrid hydrogel. Hybrid hydrogels can be tailored to have specific properties, such as printability, mechanical strength, and bioactivity, by adjusting their composition and crosslinking conditions.
Properties of Hydrogels for 3D Bioprinting:
Printability
Printability is a critical property of hydrogels for 3D bioprinting, as it determines the ability of the hydrogel to be extruded through a nozzle and maintain its shape upon deposition. Factors that influence the printability of hydrogels include their viscosity, gelation kinetics, and crosslinking mechanism. Hydrogels with appropriate printability can be used to fabricate complex, high-resolution tissue constructs with precise control over their geometry and cellular composition.
Mechanical Properties
The mechanical properties of hydrogels, such as their stiffness and toughness, are important for 3D bioprinting, as they determine the ability of the printed tissue constructs to maintain their shape and withstand mechanical forces during and after the printing process. Hydrogels with tuneable mechanical properties can be used to mimic the mechanical properties of various native tissues, such as soft tissues like cartilage and stiffer tissues like bone.
Biocompatibility and Bioactivity
Biocompatibility and bioactivity are essential properties of hydrogels for 3D bioprinting, as they determine the ability of the printed tissue constructs to support cell adhesion, proliferation, and differentiation. Hydrogels with high biocompatibility and bioactivity can be used to fabricate functional tissue constructs that can integrate with the host tissue and promote tissue regeneration.
Applications and Advancements
3D printed biomaterials are being developed for use in several application areas.
Tissue Engineering
One of the primary applications of hydrogels in 3D printing is tissue engineering, where they serve as bioinks to create scaffolds that support cell growth and tissue formation. Hydrogels can be tailored to mimic the extracellular matrix (ECM) of various tissues, providing a suitable environment for cell adhesion, proliferation, and differentiation. Researchers have successfully used hydrogel-based bioinks to 3D print a variety of tissue types, including cartilage, bone, skin, and blood vessels.
Drug Delivery
Hydrogels can also be used in 3D printing for drug delivery applications. By incorporating therapeutic agents, such as small molecules, proteins, or nucleic acids, into hydrogel-based bioinks, researchers can create customized drug delivery systems with controlled release profiles. This approach allows for the fabrication of patient-specific implants or devices that can provide localized and sustained drug release, potentially improving treatment outcomes and reducing side effects.
Soft Robotics
Another more recently emerging application of hydrogels in 3D printing is the development of soft robotic systems. Hydrogels' unique mechanical properties, such as flexibility and responsiveness to external stimuli, make them ideal materials for creating soft actuators and sensors. Researchers have demonstrated the fabrication of hydrogel-based soft robots capable of mimicking the movements of living organisms, such as octopuses and jellyfish, with potential applications in underwater exploration, environmental monitoring, and biomedical devices.
Hydrogels have shown great promise in various 3D printing applications, including tissue engineering, drug delivery, and soft robotics. Their unique properties, such as high water content, biocompatibility, and tuneable mechanical properties, make them ideal candidates for these applications. As research in this field continues to advance, we can expect to see even more innovative and impactful uses of hydrogels in 3D printing.
IMAGE credit Phillip Ezze