Shear joy: Bioinks enable 3D bioprinting
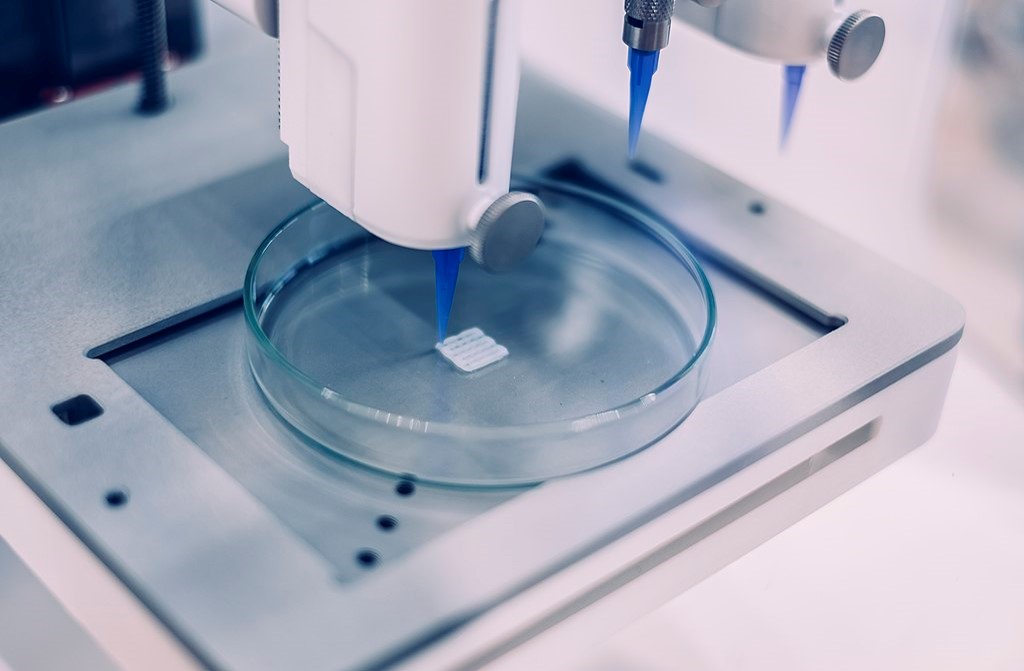
Interest in the field of three-dimensional (3D) bioprinting has increased enormously over the past ten years, thanks in no small part to its ability to precisely place different biomaterials, biomolecules and cell types together in a predefined position to generate printed composite architectures.
3D bioprinting combines the fields of computer and materials science, developmental biology and stem cells to allow researchers to create complex bio-hybrid structures for use in various applications. This has meant it has allowed the study of organs and tissues in the field of tissue engineering to be taken to the next level and has also allowed new models to be generated to aid the study of disease pathogens.
One of the most important components of bioprinting, however, is the bioink – a mixture of bioactive molecules, biomaterials and cells that are used to create the printed article.
What are Bioinks?
Bioinks contain biomaterials and living cells that mimic the extracellular matrix environment and support cell adhesion, differentiation and proliferation after printing. In contrast to more traditional 3D printing materials, bioinks must:
- Contain bioactive components that are non-toxic and that can be modified by the cells after printing
- Contain mild cross-linking or gelation conditions
- Have print temperatures that do not exceed physiological temperatures
What are the Current Bioprinting Techniques?
There are six main types of bioprinting techniques used currently, and these are:
- Extrusion-based bioprinting is the series of techniques used to manufacture objects through the sequential delivery of material in a layer-by-layer manner per computer-aided design (CAD) data
- Inkjet-based bioprinting is a non-contact printing technique where droplets of dilute solutions are dispensed through a microvalve, piezoelectric or thermal process
- Stereolithography (SLA) bioprinting uses light-reactive thermoset materials (resin) which are exposed to certain wavelengths of light in order to join molecular chains together, polymerising oligomers and monomers into solidified flexible or rigid geometries
- Laser-based bioprinting uses a laser as the energy source to deposit biomaterials onto a substrate
- Fused-deposition modelling (FDM) bioprinting is a form of extrusion printing where the desired material is passed through a heating tool in solid form as a filament, the heating tool melts the filament which can then be deposited in a layer-by-layer fashion as per the CAD model
- Selective laser sintering (SLS) bioprinting is an additive manufacturing technique that uses a laser as the power source to sinter powdered material, aiming the laser automatically at points in space as defined by a 3D model, then binding the material together to create a solid structure
Any one of these methods can be used in the bioprinting process, and the one you choose will depend on the type of ink selected as well as the complexity of the final tissue structure.
Types of Bioink
There are four main types of bioink available, and these are:
- Animal-derived bioinks are able to provide adapted scaffolding systems for the structural and functional organisation of cells
- Natural bioinks possess unique properties such as biocompatibility and biodegradability, making them suitable for several tissue engineering applications
- Polymer-based bioinks possess medium to high viscosity in solutions based on concentration and undergo physical cross-linking via hydrogen bonding
- Peptide-based bioinks, such as Cell Guidance Systems PeptiInks®, have been specifically developed for bioprinting and advanced 3D cell culture applications as they form a nanofibrous network that mimics the extracellular matrix
When you are choosing a bioink, there are various things you should keep in mind, including:
- Bioprintability – how controllable is the formation of well-defined droplets and the morphology and shape fidelity of the deposited building blocks?
- Biocompatibility with live cells – how compatible is the material to living tissue? Will it produce an immunological or toxic response when exposed to the body or bodily fluids?
- Biodegradation – how likely is it that the material will break down when exposed to microorganisms?
- In situ gelation – will the solid model change to a gel form under certain physiological conditions?
- Shear-thinning – will the viscosity of the bioink decrease when shear stress is applied?
- Viscoelasticity – if the bioink has life-like viscoelasticity it can lead to better tissue formation
The Pros and Cons of Bioinks
There are pros and cons for each type of bioink, as with most other materials. Natural bioinks, for example, are not harmful or toxic to living tissue (biocompatible) as well as being functional. However, there tends to be significant batch-to-batch variation when used meaning results are not translatable, and they cannot be scaled.
IMAGE credit Phillip Ezze