Stabilizing and storing exosomes
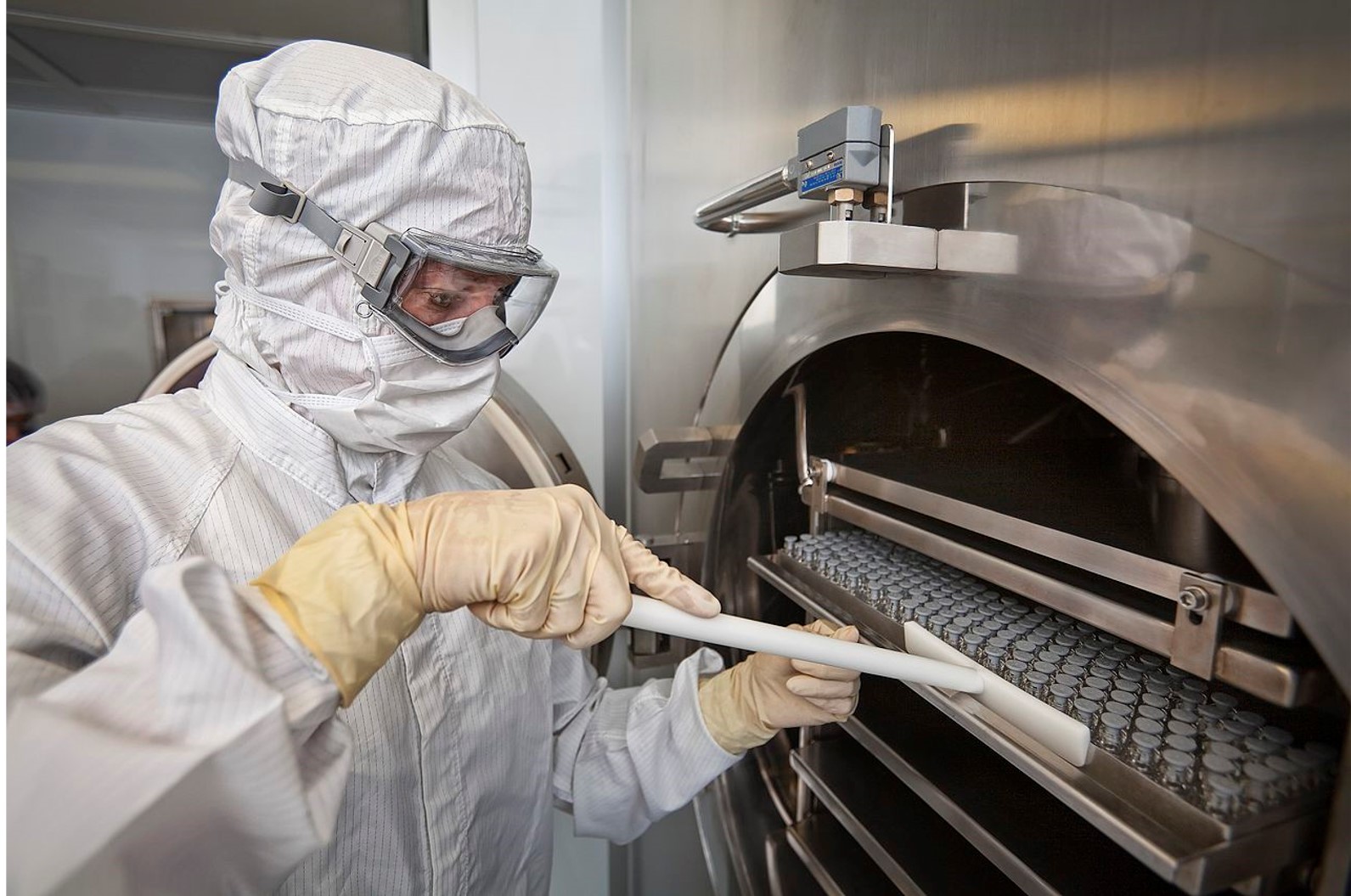
Exosomes represent a potential sweet spot between small molecules, biologics, and cell therapies for a future as highly potent multifaceted biopharmaceuticals. Studies that are leading towards clinical applications have raised expectations that exosomes will eventually provide treatment for a wide range of orphan diseases. From basic research to clinical application, the ability to stably store exosomes whilst maintaining their unique characteristics is fundamental to their research and therapeutic development.
As with cells and other cell-derived products, the development of exosomes for clinical applications still faces many challenges. In addition to preparing and characterising exosomes from cell supernatants, many hurdles still exist in the maintenance of biological activity during transport and storage.
Get your EVs freeze-dried
Isolated exosomal samples are routinely stored at -80°C but are susceptible to damage associated with low temperatures. Changes in viability, morphology and altered bioactivity during long-term storage at low temperatures are often reported. For instance, compared to freshly isolated exosomes, the diameter of bronchoalveolar lavage fluid (BALF) exosomes increases by 25% when stored at −80°C due to multilamellar structure formation. A long-term storage period (2 years) also degraded the exosomal miRNA, even when samples were frozen at −80°C.
Cryopreservation is well established as a simple and practical means for storing and transporting exosomes. Cryopreservation involves the use of liquid nitrogen (-196°C) or the gas phase above the liquid nitrogen at (-150°C) for freezing and storing the biomaterials to the temperatures below that required for biochemical reactions.
By using liquid nitrogen for long-term storage, cryopreservation has been shown to maintain a high level of viability and functionality for the exosomes. Freezing of the exosomes can be achieved through two different approaches, namely slow freezing and vitrification.
Both slow freezing and vitrification involve the solidification of the aqueous milieu of the cell or tissue into a noncrystalline glassy phase. However, as an ultrarapid ice-free cooling technique that takes only a few seconds, vitrification allows for better control of the cooling process and has been shown to yield better results than slow-freezing methods.
The main limitation in the process of cryopreservation is the inevitable water-to-ice phase transition during the freezing-thawing procedures. Often termed as cryoinjury, the formation, growth and recrystallisation of ice crystals associated with low temperatures and osmotic imbalance usually lead to cells sustaining functional and morphological ice damage. Thus, to overcome cryoinjury, cryopreservation is commonly associated with the addition of cryoprotectants
The main principle of cryoprotectants is by increasing the solute concentration in cells — impacting the kinetics of ice nucleation and allowing regulated extracellular, instead of intracellular, ice growth. There are two main classes of cryoprotectants based on their permeability and molecular weight, namely the penetrating and non-penetrating cryoprotectants.
By definition, penetrating cryoprotectants works intracellularly by penetrating the lipid bilayer membrane, as characterised by their low molecular weight (glycerol and DMSO.
On the contrary, non-penetrating cryoprotectants work extracellularly through the formation of hydrogen bonds with the biomolecules — establishing a stable glassy matrix that coats the outside of the biomolecules for cryoprotective effects. Non-penetrating cryoprotectants are usually polymers with high molecular mass (180-594 Da), such as Polyethene glycol (PEG), polyvinylpyrrolidone (PVP) and non-reducing disaccharides.
Among the currently characterised cryoprotectants, trehalose is well-known as the safest and best cryoprotectant for different kinds of cells. Trehalose deconstructs the tetrahedral hydrogen bond network of water — slowing the dynamics of water dramatically and physically shields the biomolecule against crystallisation for an additional, slower, relaxation process in the dynamics of water when the temperature decreases.
If there were no limit to the concentration of cryoprotectants that could be used, cells would be preserved perfectly. However, excessively high concentrations of cryoprotectants can be toxic. Thus, to achieve optimal cryopreservation and prevent cryoinjury, it’s noteworthy that the concentration of cryoprotectants should be adequately maintained at the appropriate balance.
A combination of both penetrating and non-penetrating cryoprotectants have also been shown to exhibit less toxicity and be more effective than single-agent cryoprotectants. Some good examples of cryoprotectant combinations include a mixture of formamide-DMSO, sugar-DMSO, ethylene glycol-propanediol, etc. Other alternatives of chemical ice-inhibition molecules, as summarised in this review, may also serve as promising candidates for preventing cryoinjury in exosomes.
Another widely used technique for exosomes preservation is lyophilisation, commonly achieved through freeze-drying and/or spray drying. Lyophilised materials can be stored at room temperature — eliminating the risk of damages to samples during freezing/thawing and the need for cold-chain-dependent transportation and storage.
Protocols for exosomes lyophilisation using trehalose and mannitol as cryoprotectants have been reported. Preliminary studies on the morphology and bioactivity of the lyophilised exosomes have been conducted minimally on BMSC-derived exosomes and CPP-modified exosomes.
As critical questions around scalable manufacturing and long-term preservation including lyophilization are answered, exosome-based therapeutics may offer a more affordable form of regenerative medicine, thereby increasing market penetration and patient access. Collectively, various storage factors and the different aspects of the exosomes isolated from different sources and experimental techniques should be further explored for standardisation and optimisation of cryopreservation for routine use.
While regulatory hurdles for this new class of biologics for clinical approach remain a challenge to be met, the relative stability of exosomes will likely see a significantly expedited path to regulatory approval.
IMAGE: Integrity Bio, pharmaceuticals undergoing lyophilization.
Learn more about powerful technologies that are enabling research: