Cancer wars: the return of the cytokine
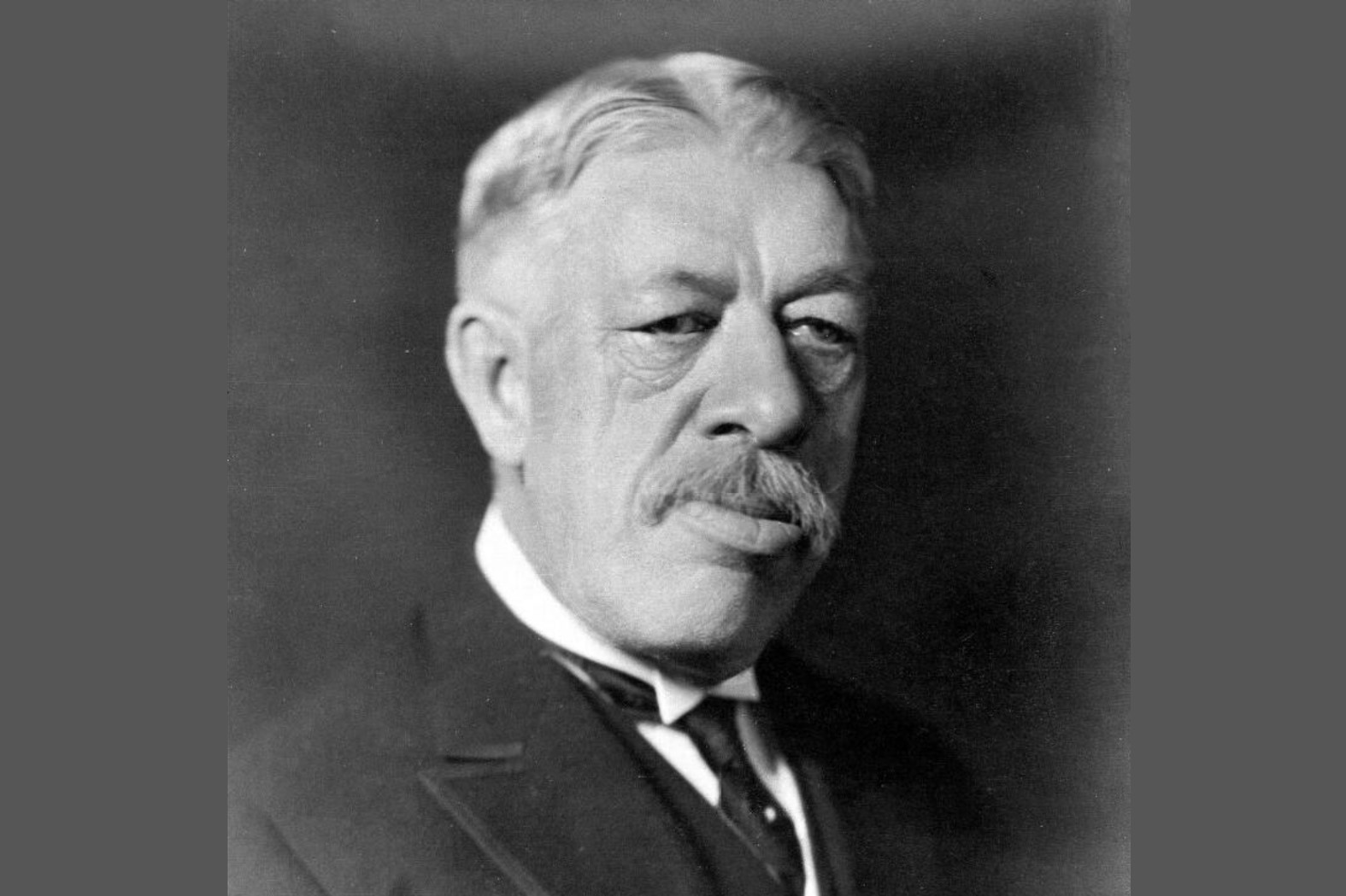
In the 1890s, William Coley (pictured) pioneered techniques that boost immune activity against cancer by injecting pathogens into cancer patients to stimulate their immune system. The modern emergence of onco-immunotherapy began with the therapeutic development of cytokines. These messenger proteins modulate both innate and adaptive immunity. Although they have been overshadowed by checkpoint inhibitors for the last decade, there is renewed interest in targeting and harnessing cytokines for cancer immunotherapy.
A role for cytokines in cancer treatment first gained prominence when high-dose IL-2 was approved in 1992 for the treatment of metastatic renal cell carcinoma and subsequently melanoma. This breakthrough was soon followed by the approval of recombinant IFN-α for the adjuvant treatment of high-risk melanoma patients and several other refractory malignancies. These early milestones spurred further research that has led to the identification of a variety of cytokines and cytokine receptors as either cancer treatments or targets respectively.
Cytokines have been shown to act on every phase of the cancer immunity cycle by improving antigen priming, increasing the number of effector immune cells in the tumour microenvironment and enhancing their cytolytic activity. Preclinical experiments to enhance the growth inhibitory and immunostimulatory effects of different pro-inflammatory cytokines (VEGF, GM-CSF, IFN-γ, IL-7, IL-12, IL-15 and IL-21) and inhibit the tumour-promoting actions of anti-inflammatory cytokines (TNF, IL-1β and IL-6) have demonstrated their therapeutic potential. However, clinical trials almost always fail to fulfil the promise of efficacy seen in preclinical experiments. IFN-α and IL-2 remain the only FDA approved cytokines for cancer therapy. Other approved drugs, such as EPO, address side effects and not the cancer itself. Moreover, therapeutic cytokines have largely been superseded by other safer (less toxic) and more efficacious immunotherapeutic agents, notably immune checkpoint inhibitors.
The immune system is also coupled with a multitude of fail-safes, negative feedback loops and immune checkpoints that enable precision control which provides opportunities for cancer cells to disengage and/or shut down an immune response. One of the issues with cytokines is their pleiotropic, redundant and often conflicting nature caused by a chronic tug-of-war between the highly regulated nature of the immune system and the adaptability/variability of cancer. For example, IL-2 activates immune-suppressive T-regulatory cells (T-regs) as well as T cells. Some companies are developing second-generation IL-2 therapies which do not stimulate T-regs. Similarly, the directed evolution of yeast has been used to develop a “decoy-resistant” IL-18 variant that attaches specifically to IL-18R, instead of the ‘decoy’ IL-18BP present on cancer cells, to enable its immune-activating functions.
A further limitation of using cytokines as therapeutics is their instability. Cytokines possess a short serum half-life that necessitates high doses and frequent systemic administration for them to achieve therapeutic efficacy. This increases the risk of severe dose-limiting toxicity. The handling/storage of cytokines (freeze-thaw cycles, storage time, etc.) also affect their physicochemical stability and may cause denaturation/variations of crucial properties during applications.
Given these issues, one key goal for clinical development of cytokines for cancer immunotherapy has been to minimise plasma exposure while maintaining efficacy, and, therefore, reduce overall toxicity. One strategy to achieve this is to modify the pharmacokinetic properties of cytokines through genetic engineering to increase stability and bioactivity. Sustained-release cytokine formulations such as PODS reduce issues associated with short half-lives. PODS also have the potential for passive targeting of cytokines to cancer using macrophages as Trojan horses.
Genetic fusion of cytokines with other targeting/functional proteins (bacterial toxins, antigens, antibodies etc.) to introduce new functionalities and modulate their pharmacokinetic profiles can also enhance the resulting protein complex. Cytokine fusion proteins promise superior efficacy and improved biologic manufacturability, due to their increased size and lowered interstitial transport rate, compared to their original counterparts.
Another strategy uses bioconjugation and transport of cytokines in cellular vesicles: By exploiting the properties of different biomaterials and micro-/nanoparticles (PEG, polymer matrices, lipid-based microparticles, etc.), researchers can achieve a variety of modifications including the material composition, charge, shape and flexibility to localise cytokine responses, increase local drug concentrations, enhance efficacy and reduce systemic off-target proinflammatory effects. One of the most recent additions to this field of research includes the tumour-derived exosome delivery approach to enhance cytokine-based cancer treatment.
The tumour microenvironment is complex and rapidly evolves in response to therapy. The challenge of demonstrating therapeutic effects beyond animal models presents a major challenge to the development of effective cytokine therapeutics. Important differences in vascularisation, immune infiltration, cellular makeup, receptor expression and cytokine responses between human and animal models can have significant effects on the delivery/efficacy of cytokine treatment, further hindering the clinical translation of cytokine-based immunotherapy.
In addition to single-agent treatments, one of the more promising areas for cytokine immunotherapeutics is combination therapies using cytokines as adjuvants for vaccines, alongside checkpoint inhibitors, and with surgery, chemotherapy and radiotherapy. There is strong initial clinical evidence that lower systemic toxicities may be observed when administered at longer infusion rates to sustain lower blood levels. For example, administration of IL-2 concurrent with or after IFN-α therapy can prevent toxicities observed during individual subsequent administrations.
IMAGE: William Coley Credit: Wellcome Trust archives
Learn more about powerful technologies that are enabling research: