Green fingers: Plant scaffolds for body parts
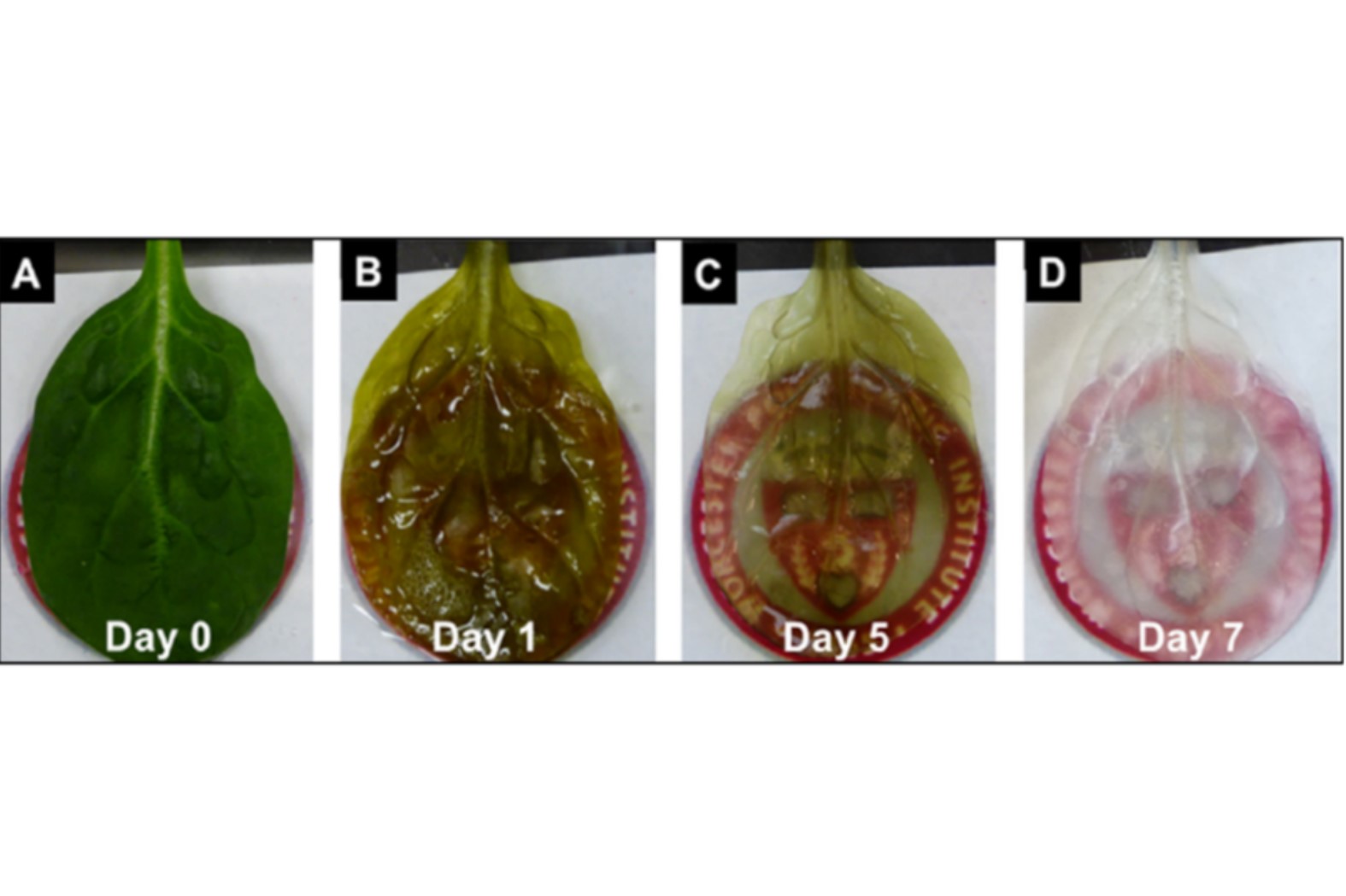
Wood, bamboo and other plant-derived materials are widely used to provide structural integrity for buildings. It turns out that plant-derived scaffolds can also be used, on a much smaller scale, to support the culture of cells grown in 3D. Importantly, as well as providing structure, plant structures can provide vasculature, on a similar scale to our own, enabling nutrients and signalling molecules to be carried to cells that are distant from the surface.
Cell culture is mostly performed in 2D on flat surfaces. This produces cells that in many respects are far removed from their in vivo counterparts. Over recent decades, a 3rd dimension has been added to cell culture. Notably, organoids have been generated to model a wide range of tissues. But organoids' size is limited by rates of diffusion of gases, nutrients, signalling molecules and metabolites to and from the culture media. Larger 3D in-vitro structures that can more accurately model in-vivo tissue require structured scaffolds with vasculature.
3D culture was established using animal-derived polymers (e.g. Matrigen, collagen, gelatin). These hydrogels are still widely used, but they have some significant limitations: Their animal origin can lead to variability safety and ethical concerns, particularly with the availability of non-animal alternatives. Consequently, plant-derived (e.g. cellulose, alginate) and synthetic (e.g. peptide) hydrogel scaffolds are increasingly used. However, all of these scaffold materials are essentially amorphous hydrogels, lacking complex structure or vasculature.
Bioprinting can be used to directly print cells in hydrogel scaffolds to direct the development of complex structures. In addition, acellular scaffolds can be printed onto which cells are subsequently seeded. Printing such 3D structures at the level of definition required for vasculature is within the sites of at least one company but only on a small scale. To generate larger scaffolds with vasculature, a simpler approach is to use existing living structures and decellularize them. This approach was used to generate tracheal transplants using decellularized donor trachea colonized with autologous cells derived from the patient.
More recently, decellularized plant material has emerged as a relevant alternative. The plant materials are subjected to a decellularization process in which chemoenzymatic methods are used to remove cells. The extracellular matrix is preserved to provide structural and biomechanical support. This decellularized scaffold can then be repopulated with specific cells to produce tissue-engineered constructs for various biomedical applications.
Andrew Pelling’s lab in Ottawa, Canada is one of the pioneers in this field. Their first proof-of-concept study demonstrated how decellularized apple hypanthium can be used as a substrate for 3D cell culture to proliferate mammalian cells. This feat has been repeated by other labs with different mammalian cells, either cell lines or primary cells, used to repopulate scaffolds generated from numerous plants, including bamboo, parsley stems, palm fibres, and others.
Plant decellularized scaffolds offer many biochemical, physical/architectural and practical benefits. Since plant tissue is primarily built from cellulose, plant-based scaffolds are hydrophilic, durable, biocompatible and non-immunogenic. Additionally, plant-based scaffolds possess the requisite architecture, including a high surface/volume ratio, interconnected porosity and an excellent vasculature.
If desired, the plant’s vein network can be recellularized with endothelial cells to modulate the vascular component. In comparison to synthetic microchannel development techniques, decellularized vascular bundle of plants provide a low cost, highly accessible and easy-to-use material to study cellular behaviour.
Mechanosensing is critical to cellular function and ultimately, tissue formation. Plant-based scaffolds match the stiffness range of most human tissues. In addition, plant scaffolds are pliable and can be easily manipulated using either biofunctionalisation or chemical crosslinking to form a wide array of topographical and biomechanical environments.
Prior to seeding of cells onto the scaffold, vegetal tissue is often treated with ECM proteins (e.g. collagen, fibronectin) and other crosslinking agents (glutaraldehyde) to functionalize the surfaces and enhance cell adhesion/proliferation or modulate relevant biomechanical properties (stiffness, tensile properties, etc.) to mimic the mechanical properties of the tissue being reconstructed. These coatings provide mammalian biochemical cues that may drive appropriate cell behaviour. Cytokines may also be supplemented locally by pre-treatment of scaffolds with sustained-release proteins by PODS.
With all these advantages, decellularized plants are gaining popularity. Their potential is illustrated by a study of decellularized spinach leaves to model the cardiac environment. The inner vascular network of the plant and the surface of the leaf were recellularized with human endothelial cells and cardiomyocytes, respectively. The 50–200 µm diameter perfusable microvasculature, overcame the 100–200 μm oxygen diffusion limit of other 3D scaffolds.
Significant hurdles to the widespread adoption of plant vasculature for 3D cell culture. The recellularization of these delicate biological microfluidic systems through the vasculature can be challenging. The high flow rate generated via syringe injection leads to increased internal pressure that may damage the scaffold. The use of a flow controller, such as a syringe pump, may help to maintain an adequate flow rate—but the presence of hydrophobic lignin polymers found in vascular plant cell walls and the inherent porosity of the scaffold ultimately discourages cell attachment and promotes cell leaking, respectively.
IMAGE Spinach leaf Int. J. Mol. Sci. 2021, 22(22), 12347; https://doi.org/10.3390/ijms222212347