Your gene therapist will see you now
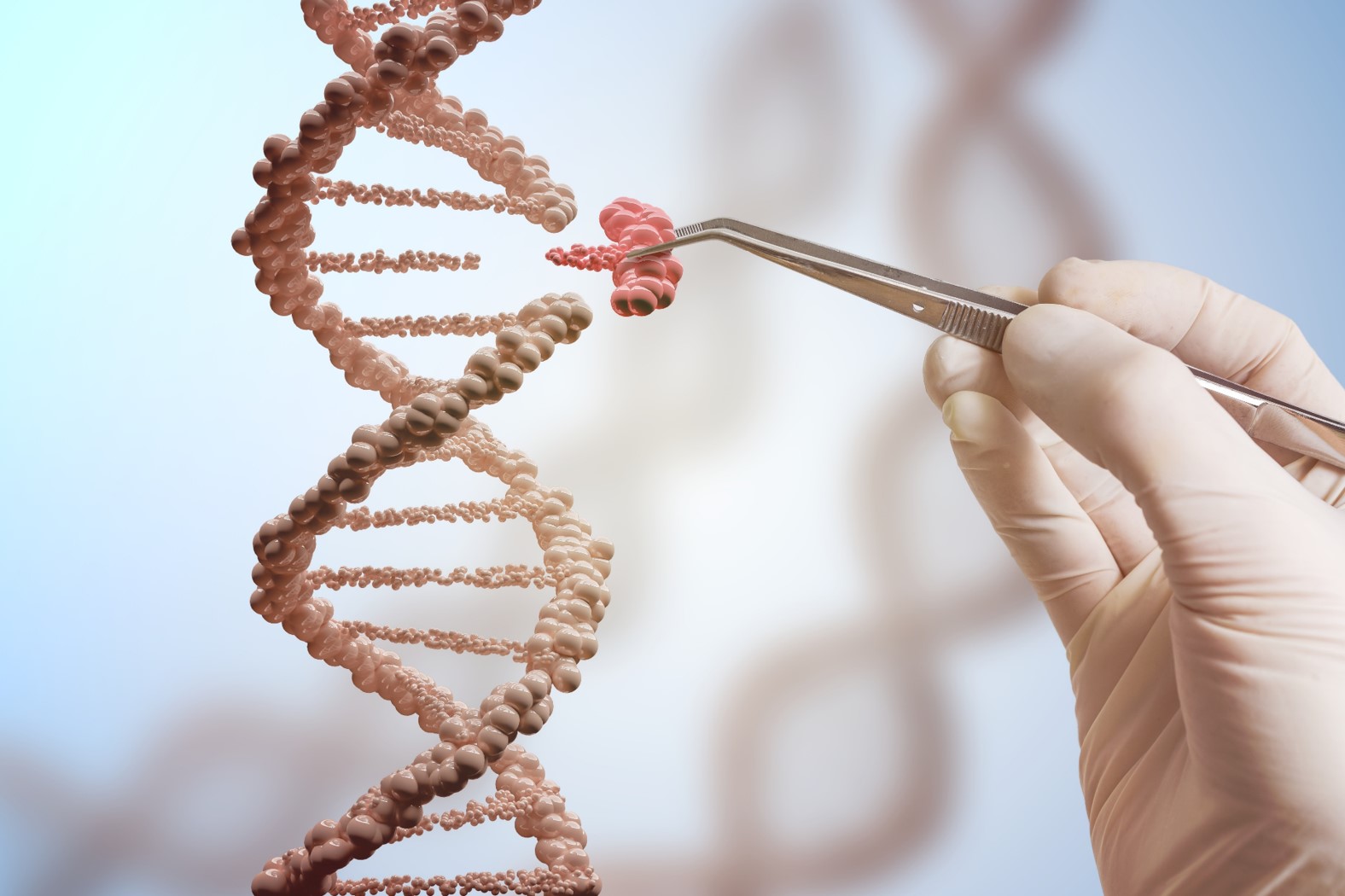
Just a decade ago, for the millions of people living with one of the thousands of rare genetic diseases, there seemed little hope that an effective treatment could be developed. One of the main obstacles is the massive costs of drug development; for many rare genetic diseases, there aren't even enough patients to conduct a formal clinical trial. But changes to the regulatory framework and the development of gene therapy platform technologies that, once proven safe, may be rapidly turned to a wide number of disparate diseases, are offering hope.
Genetic therapy uses DNA or RNA molecules as drugs to modify the structure or regulate the expression levels of defective target genes. Now proven in the clinic, as the genetic therapy field matures, more gene therapy platform technologies are being developed. These are able to modulate every aspect of protein expression control, from altering the composition of chromosomal DNA right up to interventions at the point where amino acids assemble into proteins on ribosomes.
Genomic DNA editing can be used to alter the structure and function of proteins to address the root cause of many genetic disorders. The advent of CRISPR/Cas9 and related technologies have made DNA editing routine, transforming the field. But this technique suffers from low levels of off-target editing and alternatives to CRSIPR/Cas9 have emerged which offer improvements in the safety/specificity of genomic editing. Despite this, genomic editing is always likely to suffer from potentially irreversible/deleterious impacts. An answer may lie in the exploration of alternative methods to correct disease-causing variants. One such promising area is the development of RNA editing.
RNA therapy can be achieved using naturally occurring ADAR enzymes to create sequence-specific nucleotide edits of target mRNAs with high specificity. The technique has potentially fewer side effects, is easier to deliver and offers more versatility when compared to DNA-based therapies. The majority of RNA therapeutics in development utilize synthetic noncoding RNA.
Antisense oligonucleotide (ASO) and small interfering RNA (siRNA) are the two most widely used RNA therapy strategies that target nucleic acids through a cellular pathway known as RNA interference, knocking down protein expression levels. Since ASOs are single-stranded, they are more cost-effective, easier to deliver in vivo than siRNA. Although RNAs suffer from short half-lives, simple chemical modifications have been developed, such as Moderna’s technology, that can improve resistance to nucleases. Delivery is another challenge for RNA. Although siRNAs are tougher to get into cells, fewer molecules are needed for the therapy to be effective. A detailed discussion of the relative merits of ASOs and siRNAs is provided in this paper.
Other types of RNA therapeutics available include miRNAs, RNA aptamers and synthetic mRNAs. miRNAs exist as either miRNA mimics that induce the expression of proteins and/or miRNA inhibitors that repress the translation of that particular mRNA. RNA aptamers are single-stranded RNA, DNA or RNA-DNA hybrids designed to act through three main mechanisms of action with high affinity/specificity. Injection of synthetic mRNAs that encode certain peptides/proteins, either as adjuvants or antigens, has also mediated the rapid development of particularly efficient mRNA vaccines over the last couple of years.
A technology that has recently been garnering attention is SINEUP, being developed by Transine Therapeutics. SINEUPs are essentially short RNA motifs, similar to IRES elements but able act in trans which can be used to target specific genes. SINEUPs have an mRNA target binding domain and a ribosome binding domain. Once bound to the gene, they recruit ribosomes and therefore increase the efficiency of protein translation. This results in an increase in protein levels of about two- to three-fold. This may not seem like a lot, but for the hundreds, perhaps thousands of rare diseases caused by haploinsufficiency, this is exactly what is required. Because they require an mRNA target to be expressed in a cell, this helps a lot with specificity. Moreover, their subtle effects are less likely to cause significant adverse events compared to therapies which provide dramatic changes in expression levels of off-target genes.
One of the greatest challenges for gene therapy is delivery. AAV is often the method of choice, but concerns about safety and immunogenicity, and preventing re-dosing, are driving searches for alternatives. As with other viruses, AAVs exhibit tropism, a preference for infecting a particular cell type. The availability of AAVs suited to a particular target cell as well as the localized immune environment are important considerations when choosing disease targets.
The eye, in particular the retina, is probably the best candidate for RNA therapy target. As an immunologically privileged site, the eye can accommodate the antigenicity of a viral vector more readily than other organs. It is also easily accessible and is surrounded by a tight blood-retinal barrier for increased bioavailability, greater localised efficacy and minimal systemic effects. Additionally, in the retina, noncoding RNAs have been shown to play a significant functional role, regulating factors involved in apoptosis, inflammatory response, visual perception and photo-transduction, with altered levels noted in diseased states.
The idea of RNA therapy for retinal diseases is not new. Historically, the retina has played an important role in RNA therapy development, with the first approval for ASO in 1998 for intravitreal treatment of CMV retinitis. Inherited retinal dystrophies are one such category of diseases that have shown significant advancement in treatment strategies following FDA approval of the first gene therapy for a genetic disease in 2017. An overview of the current principles and development of RNA therapeutics for retinal diseases can be found here.
The development of RNA therapeutics is still faced with major hurdles. Irrespective of their therapeutic mechanism of action, the large size of some therapeutic RNAs (mRNAs), their anionic charge, and their susceptibility to ubiquitous RNases make it difficult for therapeutic RNAs to enter and function efficiently in cells. Additionally, the strong immunogenicity of exogenous RNA may cause cell toxicity and impaired translation into therapeutic proteins.
While significant innovation is still required, the many and proven advantages of RNA therapeutics are making the development of these technologies a worthwhile investment. Several dominating players in the RNA biopharma sector are emerging and new small biotech startups and academic groups with transformative ideas are propelling the limitless possibilities.
The development of RNA therapeutics is still faced with major hurdles. Irrespective of their therapeutic mechanism of action, the large size of some therapeutic RNAs (mRNAs), their anionic charge, and their susceptibility to ubiquitous RNases make it difficult for therapeutic RNAs to enter and function efficiently in cells. Additionally, the strong immunogenicity of exogenous RNA may cause cell toxicity and impaired translation into therapeutic proteins.
While significant innovation is still required, the many and proven advantages of RNA therapeutics are making the development of these technologies a worthwhile investment. Several dominating players in the RNA biopharma sector are emerging and new small biotech startups and academic groups with transformative ideas are propelling the limitless possibilities.
IMAGE: Shutterstock